Abstract
Infectious diseases are a significant burden on public health and economic stability of societies all over the world. They have been among the leading causes of death and disability and presented growing challenges to health security and human progress for centuries. Infectious diseases are generally caused by microorganisms. The routes of them entry into host is mostly by the mouth, eyes, genital openings, nose, and the skin. Damage to tissues mainly results from the growth and metabolic processes of infectious agents intracellular or within body fluids, with the production and release of toxins or enzymes that interfere with the normal functions of organs and/or systems [1]. Advances in basic science research and development of molecular technology and diagnostics have enhanced understanding of disease etiology, pathogenesis, and molecular epidemiology, which provide basis for appropriate detection, prevention, and control measures as well as rational design of vaccine [2]. The diagnosis of infectious diseases is particularly critical for the prevention and control of the epidemic. Here we introduce the insights and detection methods of infectious disease, aiming to provide some helps for clinical diagnosis as well as epidemic prevention and control of infectious diseases.
Introduction of human infectious diseases caused by living pathogens
Infectious diseases arise upon contact with an infectious agent. Five major infectious agents have been identified: bacteria, viruses, fungi, protozoans and parasites [3, 4]. Various factors can be identified that create opportunities for infectious agents to invade human hosts. These include global urbanization, increase in population density, poverty, social unrest, travel, land clearance, farming, hunting, keeping domestic pets, deforestation, climate change, and other human activities that destroy microbial habitat [5, 6]. Human engagement in activities that interfere with ecological and environmental conditions continues, thereby increasing the risk of contact with new pathogens. These pathogens are mostly transmitted though intermediate animal hosts such as rodents [7, 8], which gain increased contact with humans as a result of environmental and human behavioral factors. In most cases, a combination of risk factors accounts for infectious disease emergence and/or outbreak of epidemic. Here we list some past emerging infectious disease epidemics and probable factors for the outbreak in Table 1.
Year | Emerging disease | Pathogenic agent | Main probable factor | Genemedi’s diagnostic antibodies and antigens |
2019 | 2019-novel-coronavirus pneumonia | 2019-nCoV/SARS-CoV-2 | Dynamic balances and imbalances, within complex globally distributed ecosystems comprising humans, animals, pathogens, and the environment. May be because of hunting and feeding on infected wild animals (viverrids) |
Antigens: Nucleocapsid (N protein), Spike(S protein), RBD, S1+S2 ECD, Envelope (E protein), 3C-like Proteinase (Mpro), RdRP(Nsp12), etc. Antibodies: N protein antibody (GMP-V-2019nCoV-NAb001~004) , Spike protein antibody (GMP-V-2019nCoV-SAb001~004) |
1976-2020 | Ebola haemorrhagic fever | Filovirus Ebola virus |
Rainforest penetration by humans/close contact with infected game (hunting) or with host reservoirs (bats)/infected biological products/nosocomial/needle spread |
Antibodies: Mouse anti-ebola virus (EV) monoclonal antibodies |
1889, 1890, 1918, 1957 |
Pandemic Influenza | Paramyxovirus influenza A |
Animal-human virus reassortment and antigenic shift |
Antibodies: Anti-Influenza A NP mouse monoclonal antibody Anti-Influenza B NP mouse monoclonal antibody Antigens: Recombinant Influenza A NP Protein (Flu A/B, His Tag) Recombinant Influenza B NP Protein (Flu A/B, His Tag) |
2003 | Severe acute respiratory syndrome (SARS) | SARS Coronavirus |
Hunting and feeding on infected wild animals (viverrids) |
|
1997 | Highly pathogenic avian influenza (HPAI) | H5N1 virus |
Animal-animal influenza virus gene reassortment; emergence of H5N1 avian influenza, extensive chicken farming |
Antibodies: Anti-Influenza A NP mouse monoclonal antibody Anti-Influenza B NP mouse monoclonal antibody Antigens: Recombinant Influenza A NP Protein (Flu A/B, His Tag) Recombinant Influenza B NP Protein (Flu A/B, His Tag) |
1996 | Haemorrhagic colitis | Escherichia coli O157:H7 |
Ingestion of contaminated food, undercooked beef, and raw milk |
|
1988 | Herpes | Herpes simplex virus 1/2(HSV-1/HSV-2) | Indirect contact transmission, saliva, liquid from herpes, blood,mother to baby at birth. |
Antibodies: Mouse anti-herpes simplex virus monoclonal antibodies Antigens: Recombinant HSV-1 antigen Protein Recombinant HSV-2 antigen Protein |
1987 | Rift Valley fever (RVF) | Bunyavirus RVF virus |
Dramatic increase in mosquito vector breeding sites (by dam filling); weather (rainfall) and cattle migration (guided by artificial water holes) |
Antibodies: Mouse Anti-Rift Valley Fever (RVF) Monoclonal Antibody |
1987 | Hepatitis C | Hepatitis c virus (HCV) | Blood, acupuncture, drug taking, etc |
Antibodies: Anti-HCV core antigen (HCcAg) monoclonal antibody Antigens: Recombinant HCV NS3-NS4-NS5 fusion Protein (His Tag) |
1983 | Crimean-Congo haemorrhagic fever | CCHF virus |
Ecological changes favouring increased human exposure to ticks of sheep and small wild animals |
|
1981 | Acquired immunodeficiency syndrome (AIDS) | Human immunodeficiency virus (HIV) |
Sexual contact/exposure to blood or tissues of an infected person |
Antigens: Recombinant HIV-1 GP41 Protein (His Tag), Recombinant HIV-2 GP36 Protein (His Tag) |
1976 | Malaria | Plasmodium falciparum |
Human behaviour/rainfall and drainage problems/mosquito breeding/neglect of eradication policy, economics, and growing interchange of populations |
Antibodies: Mouse Anti-malaria monoclonal antibodies |
1969 | Lassa fever | Arenavirus Lassa virus |
Hospital exposure to index case—rodent exposure |
|
1965 | Hepatitis B | Hepatitis b virus (HBV) | sexual contact, sharing needles, syringes, or other drug-injection equipment, mother to baby at birth. | Antibodies: Mouse anti-Hepatitis b virus (HBV) monoclonal antibody |
1959 | Bolivian haemorrhagic fever (BHF) | ArenavirusMachupo virus | Population increase of rats gathering food | |
1958 | Argentine haemorrhagic fever | ArenavirusJunin virus | Changes in agricultural practices of corn harvest (maize mechanization) | |
1953 | Dengue haemorrhagic fever (DHF) | Dengue viruses 1, 2, 3, and 4 |
Increasing human population density in cities in a way that favours vector breeding sites (water storage) |
|
1949 | Cervical cancer | Human papilloma virus (HPV) | Contact infection, Sexual contact | Antibodies: Mouse anti-Human papilloma virus (HPV) monoclonal antibody |
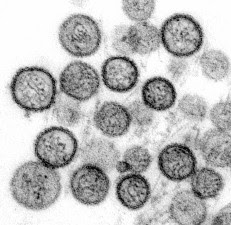
Summary
Infectious diseases are a real public health threat, outbreaks can have serious social, political, and economic effects. A complex number of factors relating to human behavior and activities, pathogen evolution, poverty, and changes in the environment as well as dynamic human interactions with animals have been found to contribute to infectious disease emergence and transmission. Aggressive research is warranted to unravel important characteristics of pathogens necessary for diagnostics, therapeutics, and vaccine development. Here we describe some strategies for the diagnosis of human infectious diseases, hoping to be helpful for clinical diagnosis and epidemic prevention and control of infectious diseases. To date, multiple diagnostic techniques have been developed. Various diagnostic tools show both significances and limitations. Conventional approaches to quantify infective viral particles are labor-intensive, time-consuming, and often associated with poor reproducibility. Immunological tests generally provide quick results, however, is quite expensive due to the requirement of antigen-specific antibody. While RT-PCR may be able to provide results within a matter of hours, it is laborious, requires a skilled operator, and is sensitive to contamination. TEM-based quantification, although highly accurate in determining the shape and the total number of viral particles, often considered time-consuming, extremely expensive and impractical for high sample numbers. Moreover, TEM sample preparation is tedious, and the technique requires sophisticated instrument and a skilled operator. To alleviate these limitations, there is still a need to develop new cost-effective analytical methods that can allow users to quickly and easily determine virus concentrations and reduce constrictions coupled with current assays. Nevertheless, any such emerging methods must be carefully evaluated in terms of their efficiency, precision and linear range. The evaluation of each diagnostic technique and approval from the FDA are necessary before practical application.
Products list: paired diagnostics grade antibodies (monoclonal antibody, mab) and antigens for infectious disease rapid test kit
Cytokine release syndrome (CRS) is an acute systemic inflammatory syndrome characterized by fever and multiple organ dysfunction.
Genemedi produces core diagnostic ingredients for test of infectious diseases and related syndrome.
GeneMedi offers paired diagnostics grade antibodies (monoclonal antibody,mab)and antigens for infectious disease rapid test kit including infection of HIV/AIDS,Hepatitis C virus(HCV), Influenza A/B,Treponema Pallidum caused Syphilis,Helicobacter pylori (H. pylori) Bacteria, Herpes simplex virus 1/2(HSV-1/HSV-2),Cytomegalovirus (CMV),rubella virus,toxoplasma gondii and so on.
All the antibodies of inflammation and CRS test are suitable for in functional ELISA, and other immunoassays in diagnostics. The antibody can act as a capture antibody and detection antibody.
Reference
SARS-CoV-2 using a colloidal gold immunochromatography assay[J]. Chinese Journal of Virology, 2020, 36(3):348-354.[25] Li H, Li YY, Zhang ZG, et al. Establishment and clinical performance evaluation of 2019 novel coronavirus antibody colloidal gold detection method[J]. Chinese Journal of Infectious Diseases, 2020, 38(3):139-144.[26] Augustine R, Das S, Hasan A, S A, Abdul Salam S, Augustine P, Dalvi YB, Varghese R, Primavera R, Yassine HM, Thakor AS, Kevadiya BD. Rapid Antibody-Based COVID-19 Mass Surveillance: Relevance, Challenges, and Prospects in a Pandemic and Post-Pandemic World. J Clin Med. 2020 Oct 21;9(10):3372. doi: 10.3390/jcm9103372. PMID: 33096742; PMCID: PMC7589650.[27] LI Jia-jun, ZHENG Xiao, et al. Novel Coronavirus and Research Progress of Related Clinical Detection Methods[J]. Biotechnology Bulletin, ISSN 1002-5464, CN 11-2396/Q.[28] Timothy M Uyeki, Henry H Bernstein, et al. Clinical Practice Guidelines by the Infectious Diseases Society of America: 2018 Update on Diagnosis, Treatment, Chemoprophylaxis, and Institutional Outbreak Management of Seasonal Influenza, Clinical Infectious Diseases, Volume 68, Issue 6, 15 March 2019, Pages e1–e47, https://doi.org/10.1093/cid/ciy866[29] Anestad G. Surveillance of respiratory viral infections by rapid immunofluorescence diagnosis, with emphasis on virus interference. Epidemiol Infect. 1987; 99:523-31[30] Daisy J, Lief F, Friedman H. Rapid diagnosis of influenza A infection by direct immunofluorescence of nasopharyngeal aspirates in adults. J Clin Microbiol. 1979; 9:688-92[31] Johnson J, Higgins A, Navarro A, HUANG Y, Esper F, Barton N, et al. Subtyping influenza A virus with monoclonal antibodies and an indirect immunofluorescence assay. J Clin Microbiol. 2012; 50:396-400[32] Fauvel M, Ozanne G. Immunofluorescence assay for human immunodeficiency virus antibody: investigation of cell fixation for virus inactivation and antigen preservation. J Clin Microbiol. 1989; 27:1810-3[33] Klespies S, Cebula D, Kelley C, Galehouse D, Maurer C. Detection of enteroviruses from clinical specimens by spin amplification shell vial culture and monoclonal antibody assay. J Clin Microbiol. 1996; 34:1465-7[34] Coffin S, Hodinka R. Utility of direct immunofluorescence and virus culture for detection of varicella-zoster virus in skin lesions. J Clin Microbiol. 1995; 33:2792-5[35] Caviness A, Oelze L, Saz U, Greer J, Demmler Harrison G. Direct immunofluorescence assay compared to cell culture for the diagnosis of mucocutaneous herpes simplex virus infections in children. J Clin Virol. 2010; 49:58-60[36] Pouletty P, Chomel J, Thouvenot D, Catalan F, Rabillon V, Kadouche J. Detection of herpes simplex virus in direct specimens by immunofluorescence assay using a monoclonal antibody. J Clin Microbiol. 1987; 25:958-9[37] Liu I, Chen P, Yeh S, Chiang Y, Huang L, Chang M, et al. Immunofluorescence assay for detection of the nucleocapsid antigen of the severe acute respiratory syndrome (SARS)-associated coronavirus in cells derived from throat wash samples of patients with SARS. J Clin Microbiol. 2005; 43:2444-8[38] https://fineartamerica.com/featured/6-vaccinia-virus-infected-cell-dr-dan-kalman.html[39] Schochetman G, Epstein J, Zuck T. Serodiagnosis of infection with the AIDS virus and other human retroviruses. Annu Rev Microbiol. 1989; 43:629-59[40] Damen M, Zaaijer H, Cuypers H, Vrielink H, van der Poel C, Reesink H, et al. Reliability of the third-generation recombinant immunoblot assay for hepatitis C virus. Transfusion. 1995; 35:745-9[41] Kong Q, Xue C, Ren X, Zhang C, Li L, Shu D, et al. Proteomic analysis of purified coronavirus infectious bronchitis virus particles. Proteome Sci. 2010; 8:29[42] Ren X, Xue C, Kong Q, Zhang C, Bi Y, Cao Y. Proteomic analysis of purified Newcastle disease virus particles. Proteome Sci. 2012; 10:32[43] Nagler F, Rake G. The Use of the Electron Microscope in Diagnosis of Variola, Vaccinia, and Varicella. J Bacteriol. 1948; 55:45-51[44] Gust I, Kaldor J, Cross G, Waugh M, Ferris A. Virus-like particles associated with a faecal antigen from hepatitis patients and with Australia antigen. Aust J Exp Biol Med Sci. 1971; 49:1-9[45] Kapikian A, Wyatt R, Dolin R, Thornhill T, Kalica A, Chanock R. Visualization by immune electron microscopy of a 27-nm particle associated with acute infectious nonbacterial gastroenteritis. J Virol. 1972; 10:1075-81[46] Johnson K, Lange J, Webb P, Murphy F. Isolation and partial characterisation of a new virus causing acute haemorrhagic fever in Zaire. Lancet. 1977; 1:569-71[47] Pattyn S, van der Groen G, Jacob W, Piot P, Courteille G. Isolation of Marburg-like virus from a case of haemorrhagic fever in Zaire. Lancet. 1977; 1:573-4[48] Chua K, Wong E, Cropp B, Hyatt A. Role of electron microscopy in Nipah virus outbreak investigation and control. Med J Malaysia. 2007; 62:139-42[49] Hyatt A, Zaki S, Goldsmith C, Wise T, Hengstberger S. Ultrastructure of Hendra virus and Nipah virus within cultured cells and host animals. Microbes Infect. 2001; 3:297-306[50] Drosten C, Gunther S, Preiser W, van der Werf S, Brodt H, Becker S, et al. Identification of a novel coronavirus in patients with severe acute respiratory syndrome. N Engl J Med. 2003; 348:1967-76[51] Reed K, Melski J, Graham M, Regnery R, Sotir M, Wegner M, et al. The detection of monkeypox in humans in the Western Hemisphere. N Engl J Med. 2004; 350:342-50[52] https://www.hantasite.com/2017/03/hantavirus-life-cycle-and-infection.html