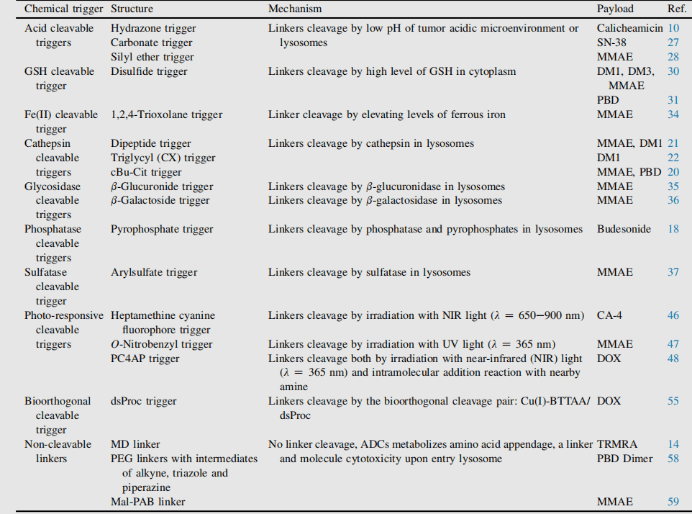
Conjugate linker is not only the molecular part forming covalent connection between antibody and small molecule payload, but also the key element with design properties in targeted drug therapy. The addition of linkers should not induce aggregation, and it is necessary to ensure acceptable PK characteristics, limit the premature release (stability) of payloads in plasma and effectively release active molecules at targeted action sites. In the process of connection, there are many conjugate companies. Connectors are divided into two types: non-cleavable linkers and cleavable linkers.1
ADC based on non-cleavable linker must be internalized, and the antibody part needs to be degraded by lysosomal protease to release active molecules. Many uncut linkers have been explored in the development of ADC. The most representative is n-succinimide-4 – (n-maleimide methyl) cyclohexane-1-carboxylate (SMCC), which is used by kadcyla.2
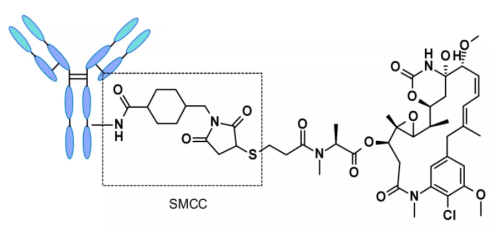
Catabolism of this structure leads to lys-smc-dm1 becoming the main tumor metabolite. In addition, drugs linked to this linker usually do not have a bystander killing/bystanerd effect, because the released catabolites have poor permeability. The current research mainly focuses on the cleavable linker.[6-7]
The use of cleavable linkers is equally feasible for the design of internalized and non internalized ADCs, because the release is triggered by the nature of the cleavage site (lysosome and / or tumor environment). Linkers can be divided into two categories: enzyme dependent and chemical (i.e. non enzyme) dependent (both have conjugate manufacturing).
Chemically dependent linker : The linker containing disulfide bond is attacked by mercaptan to release the active load. Although the reduced form of human serum albumin (HSA) in plasma is the most abundant mercaptan, its reactivity to macromolecules is very poor.8The cytoplasm also contains high levels of glutathione (GSH), a tripeptide containing sulfhydryl, which is easy to react with s-nucleophilic protein. The difference of GSH concentration in blood (micro molar range) and cytoplasm (millimolar range) and oxidative stress caused by cancer cells contribute to the preferential release of drugs in cancer cells. Linkers containing disulfide bonds are mainly related to maytansinoid payloads. The reactivity of disulfide bonds can be adjusted by steric hindrance: α- Methyl substitution significantly affects the reduction rate and resistance to mercaptan disulfide bond exchange. For example, the linker of sar-3419 obtains the best antitumor activity of spdb-DM4 through dimethyl substitution.
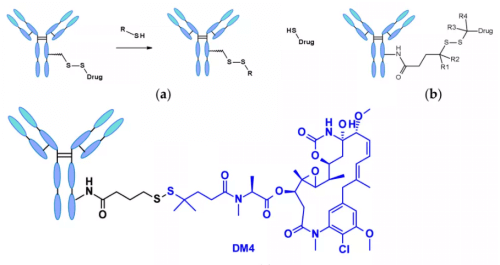
Hydrazone linkers show pH dependent stability, stable at neutral pH, and hydrolyze in acidic medium (pH < 6 of endosomes and pH < 5 of lysosomes) to form corresponding ketones and hydrazine.

The method has been successfully applied to immu-110, which contains a cleavable acyl hydrazone linker, which is formed by the reaction of the hydrazide of 4-maleimide methylcyclohexane-1-carboxylate (MCC) with the ketone group present in adriamycin.
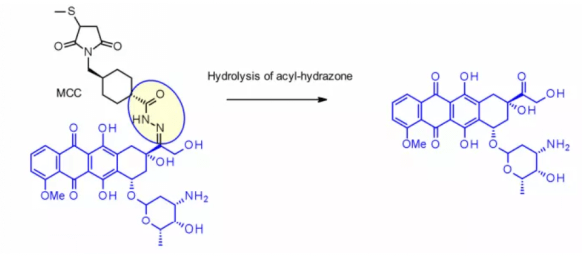
Hydrazone linkers are also often used for the payloads of the calimycin family. In this case, the release is triggered by a two-step activation process: the first step is that the acid sensitive hydrazone is hydrolyzed, and the second step is that the disulfide bond is reduced by GSH to cyclize the sulfhydryl intermediate. This linker has been used in the listed Mylotarg and besponsa, but their stability in plasma is not as stable as expected and not as attractive as other cleavable linkers.
Enzyme dependent linker: In order to limit the release of payload before internalization, so as to prevent or minimize the degradation of target cells, the protein components of lysosomes become a reasonable place to find enzymes that can degrade ADC and exist in high concentration.
Cathepsin-B
Cathepsin B is a cysteine protease, which exists in advanced endosomes and lysosomes of mammals and is overexpressed in many cancer cells. Initially, a cleavable dipeptide was used as the substrate of cathepsin B as adriamycin prodrug. This work established the dipeptide part of SAR: hydrophilic residues (citrulline or arginine) are required at P1, while lipophilic residues at P2 enhance plasma stability (phenylalanine, valine or alanine).
In addition, a self degradation spacer was introduced to promote the entry of the enzyme, thus limiting the steric hindrance of the payload: the spontaneous 1,6-elimination of p-aminobenzylcarbamate (PABA) in acidic medium, releasing carbon dioxide, p-azaquinone formamide and adriamycin. Finally, this discovery was transferred from the prodrug to the ADC field, demonstrating the antigen driven cellular activity of Val CIT and Phe Lys dipeptide linkers.4
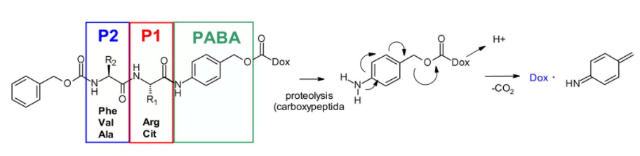
Val-Cit dipeptide is the most commonly used cleavable linker in ADCs. At present, there are up to 25 molecules in clinical stage, which may be due to its overall good plasma stability, release behavior and chemical tractability. Two approved ADC drugs (adcetris and polyvy) use the same linker MC VC PABC, which contains maleimide spacer, standard Val CIT dipeptide sequence as cathepsin substrate and PABC self degradation spacer.
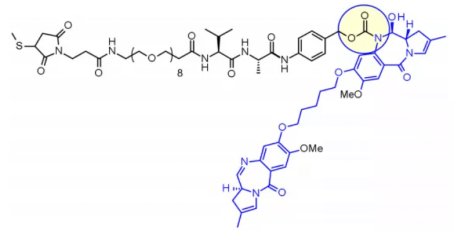
Val-Ala dipeptide is also widely used. Seven molecules are in the clinical stage. The fastest progress is loncastuximab tesirine, which includes a PEGylated spacer to balance the lipophilicity of the payload sg3199 belonging to the PBD dimer family.
Research shows that Val CIT is difficult to achieve high Dar due to precipitation and aggregation. In contrast, the Val ala linker allows Dar up to 7.4 with limited aggregation (< 10%). Compared with Val CIT, Val ala has lower hydrophobicity, which explains why this linker is excellent in lipophilic payload (such as PBD dimer). Val ala linkers of seven clinical candidate ADCs are connected to PBD.
Some studies have compared the structure of Val CIT and val ala dipeptides with the payload connection of MMAE. In the case of non internalized antibodies, Val CIT and val ala linkers bound to engineered cysteine showed similar characteristics and better performance than Val Lys and val Arg analogues. In the case of anti HER2 ADC with random cysteine binding, Val ala showed less aggregation in the high Dar structure than Val CIT. On the other hand, the two linkers showed similar buffer stability, cathepsin B release efficiency, cell activity and histopathological characteristics. Tetrapeptide Gly-Gly-Phe-Gly shows all the characteristics of stable and effective cleavable linkers, which are used by the listed ADC drug enhertu. The first trimester of enhertu is a plasma stable ADC with a dar of 7.7. Protease degradation occurs in lysosomes and dx-8951f is released. It is an effective topoisomerase I inhibitor derived from exatecan. Since the linker does not contain solubilizers, reaching such a high Dar is very considerable because it contradicts the widely established principle that high Dar conjugates may have poor pharmacokinetic characteristics. The self degradation spacer used here is simple and compact hemiamination, rather than the PABC used by Val-CIT linker.
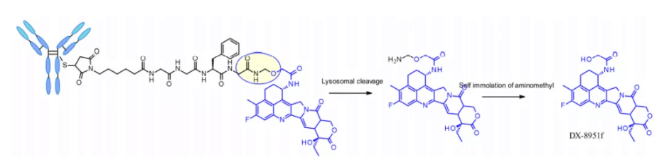
Phosphatase and pyrophosphatase
Like cathepsin, pyrophosphatase and phosphatase are hydrolases selectively expressed in lysosomes. In 2016, Merck researchers designed a linker containing phosphate and pyrophosphate to be paired with cathepsin b-sensitive Val CIT PABA to transfer glucocorticoids: phosphate / pyrophosphate partially binds between the self degrading spacer PABA and the payload. After internalization, the payload can be released in the order of cathepsin B, self degradation spacer and phosphatase (n = 1). For pyrophosphate (n = 2), another step involving pyrophosphatase may be required.
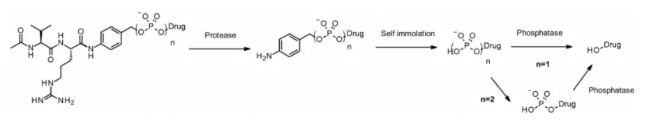
This hydrophilic and permanently charged group has the advantage of solubility. It can not only biocouple with lipophilic glucocorticoid derivatives, but also promote the purification of ADC. The residual linker in ADC is less than 0.10%. ADC containing phosphoric acid and pyrophosphoric acid has activity in vitro.
β- Glucuronidase
β- Glucuronidases are glycosidases that catalyze β- Hydrolysis of glucuronic acid residues, which are highly expressed in lysosomes and tumor stroma. Seattle genetics researchers published a groundbreaking work in 2006. Anti-CD70 ADC uses a linker containing glucuronic acid, which is attached to the self degrading spacer. This linker exhibits low levels of aggregation, high plasma stability, and strong in vivo efficacy.5
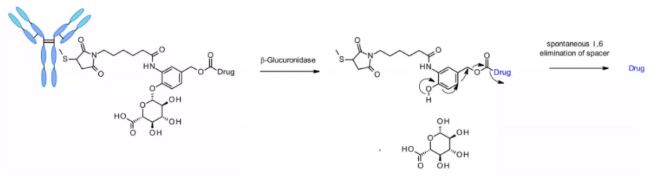
The linker is also applied to other amine containing payloads such as camptothecin analogues, sn38, ducamycin and matrine through an additional dimethylethylenediamine (DMED) self degradation spacer. Release sequence from hydrolysis β- From glucuronic acid to self degradation spacer, another cyclization reaction of DMED occurs spontaneously, forming 1,3-dimethylimidazoline-2-one, and finally releasing hydroxyl containing drugs. Due to the hydrophilicity of linker, compared with cathepsin sensitive linker, this technology makes the preparation of ADC DAR = 8 easier.

β- Galactosidase
Recently, a method of using β- Galactosidase cleaves the ADC of the linker, which contains the PEG10 spacer. The spacer was substituted by nitro to improve the self degradation rate. analogy β- Glucuronidase linker, whose dissociation mechanism involves hydrolysis β- Galactosidase moiety, which imparts hydrophilicity to chemical precursors. Another advantage is β- Galactosidase exists only in lysosomes, and β- Glucuronidase is expressed in lysosomes and in the microenvironment of solid tumors. Studies have shown that in the context of anti-HER2 ADCs releasing MMAE, it contains β- The ADC of galactosidase linker is more effective than t-dm1 in vitro and in vivo.
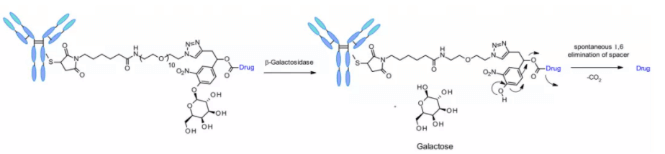
Sulfatase
Recently, there have been connectors cleaved by sulfatase, which is overexpressed in several cancer types and shows potential selectivity. The study involved anti-HER2 antibodies loaded with MMAE. Compared with the classical cleavable Val-Cit and Val-Ala linkers, the sulfatase linker showed similar efficacy on HER2+ cell lines.

Product List
Cat No. | Products Name (INN Index) | INN Name | Previous Name | Target | Format | Order |
---|---|---|---|---|---|---|
Pre-Made Losatuxizumab Vedotin Biosimilar, Whole Mab Adc, Anti-Egfr Antibody: Anti-ERBB/ERBB1/ERRP/HER1/NISBD2/PIG61/mENA therapeutic antibody Drug Conjugate |
losatuxizumab vedotin |
NA |
EGFR |
Whole mAb ADC |
||
Pre-Made Lupartumab Amadotin Biosimilar, Whole Mab Adc, Anti-Lypd3 Antibody: Anti-C4.4A therapeutic antibody Drug Conjugate |
lupartumab amadotin |
NA |
LYPD3 |
Whole mAb ADC |
||
Pre-Made Mipasetamab Uzoptirine Biosimilar, Whole Mab Adc, Anti-Axl Antibody: Anti-ARK/JTK11/Tyro7/UFO therapeutic antibody Drug Conjugate |
mipasetamab uzoptirine |
NA |
AXL |
Whole mAb ADC |
||
Pre-Made Mirvetuximab Soravtansine Biosimilar, Whole Mab Adc, Anti-Folr1 Antibody: Anti-FBP/FOLR/FRalpha/NCFTD therapeutic antibody Drug Conjugate |
mirvetuximab soravtansine |
NA |
FOLR1 |
Whole mAb ADC |
||
Pre-Made Mirzotamab Clezutoclax Biosimilar, Whole Mab Adc, Anti-Cd276 Antibody: Anti-4Ig-B7-H3/B7-H3/B7H3/B7RP-2 therapeutic antibody Drug Conjugate |
mirzotamab clezutoclax |
NA |
CD276 |
Whole mAb ADC |
||
Pre-Made Naratuximab Emtansine Biosimilar, Whole Mab Adc, Anti-Cd37 Antibody: Anti-GP52-40/TSPAN26 therapeutic antibody Drug Conjugate |
naratuximab emtansine |
NA |
CD37 |
Whole mAb ADC |
||
Pre-Made Patritumab Deruxtecan Biosimilar, Whole Mab Adc, Anti-ERBB3/Erbb-3 Antibody: Anti-ErbB-3/FERLK/HER3/LCCS2/MDA-BF-1/VSCN1/c-erbB-3/c-erbB3/erbB3-S/p180-ErbB3/p45-sErbB3/p85-sErbB3 therapeutic |
patritumab deruxtecan |
NA |
ERBB3 |
Whole mAb ADC |
||
Pre-Made Pelgifatamab Corixetan Biosimilar, Whole Mab Adc, Anti-FOLH1/GCPII Antibody: Anti-FGCP/FOLH/GCP2/NAALAD1/PSM/PSMA/mGCP therapeutic antibody Drug Conjugate |
pelgifatamab corixetan |
NA |
FOLH1 |
Whole mAb ADC |
||
Pre-Made Pinatuzumab Vedotin Biosimilar, Whole Mab Adc, Anti-Cd22 Antibody: Anti-SIGLEC-2/SIGLEC2 therapeutic antibody Drug Conjugate |
pinatuzumab vedotin |
NA |
CD22 |
Whole mAb ADC |
||
Pre-Made Pivekimab Sunirine Biosimilar, Whole Mab Adc, Anti-Il3Ra Antibody: Anti-CD123/IL3R/IL3RX/IL3RY/hIL-3Ra therapeutic antibody Drug Conjugate |
pivekimab sunirine |
NA |
IL3RA |
Whole mAb ADC |
||
Pre-Made Polatuzumab Vedotin Biosimilar, Whole Mab Adc, Anti-Cd79B Antibody: Anti-AGM6/B29/IGB therapeutic antibody Drug Conjugate |
polatuzumab vedotin |
NA |
CD79B |
Whole mAb ADC |
||
Pre-Made Praluzatamab Ravtansine Biosimilar, Whole Mab Adc, Anti-Alcam Antibody: Anti-CD166/MEMD therapeutic antibody Drug Conjugate |
praluzatamab ravtansine |
NA |
ALCAM |
Whole mAb ADC |
||
Pre-Made Rolinsatamab Talirine Biosimilar, Whole Mab Adc, Anti-Prlr Antibody: Anti-HPRL/MFAB/RI-PRLR/hPRLrI therapeutic antibody Drug Conjugate |
rolinsatamab talirine |
NA |
PRLR |
Whole mAb ADC |
||
Pre-Made Rosopatamab Tetraxetan Biosimilar, Whole Mab Adc, Anti-FOLH1/GCPII Antibody: Anti-FGCP/FOLH/GCP2/NAALAD1/PSM/PSMA/mGCP therapeutic antibody Drug Conjugate |
rosopatamab tetraxetan |
NA |
FOLH1 |
Whole mAb ADC |
||
Pre-Made Rovalpituzumab Tesirine Biosimilar, Whole Mab Adc, Anti-Dll3 Antibody: Anti-SCDO1 therapeutic antibody Drug Conjugate |
rovalpituzumab tesirine |
NA |
DLL3 |
Whole mAb ADC |
||
Pre-Made Sacituzumab Govitecan Biosimilar, Whole Mab Adc, Anti-Tacstd2 Antibody: Anti-EGP-1/EGP1/GA733-1/GA7331/GP50/M1S1/TROP2 therapeutic antibody Drug Conjugate |
sacituzumab govitecan |
NA |
TACSTD2 |
Whole mAb ADC |
||
Pre-Made Samrotamab Vedotin Biosimilar, Whole Mab Adc, Anti-Lrrc15 Antibody: Anti-LIB therapeutic antibody Drug Conjugate |
samrotamab vedotin |
NA |
LRRC15 |
Whole mAb ADC |
||
Pre-Made Serclutamab Talirine Biosimilar, Whole Mab Adc, Anti-Egfr Antibody: Anti-ERBB/ERBB1/ERRP/HER1/NISBD2/PIG61/mENA therapeutic antibody Drug Conjugate |
serclutamab talirine |
NA |
EGFR |
Whole mAb ADC |
||
Pre-Made Sirtratumab Vedotin Biosimilar, Whole Mab Adc, Anti-Slitrk6 Antibody: Anti-DFNMYP therapeutic antibody Drug Conjugate |
sirtratumab vedotin |
NA |
SLITRK6 |
Whole mAb ADC |
||
Pre-Made Sofituzumab Vedotin Biosimilar, Whole Mab Adc, Anti-Muc16 Antibody: Anti-CA125 therapeutic antibody Drug Conjugate |
sofituzumab vedotin |
NA |
MUC16 |
Whole mAb ADC |
Reference
1. Su Z , Xiao D , Xie F , et al. Antibodydrug conjugates: Recent advances in linker chemistry[J]. Acta Pharmaceutica Sinica B, 2021.
2. Chen Y , Kim M T , Zheng L , et al. Structural Characterization of Cross-Linked Species in Trastuzumab Emtansine (Kadcyla)[J]. Bioconjugate Chemistry, 2016.
3. Seki H , Walsh S J , Bargh J D , et al. Rapid and robust cysteine bioconjugation with vinylheteroarenes. 2021.
4. Kostova V, Désos P, Starck JB, Kotschy A. The Chemistry Behind ADCs. Pharmaceuticals (Basel). 2021;14(5):442.
5. Kostova, V.; Désos, P.; Starck, J.-B.; Kotschy, A. The Chemistry Behind ADCs. Pharmaceuticals 2021, 14, 442.
6. Singh A P , Sharma S , Shah D K . Quantitative characterization of in vitro bystander effect of antibody-drug conjugates[J]. J Pharmacokinet Pharmacodyn, 2016, 43(6):567-582.
7. Aleksandr V. Yurkovetskiy;Natalya D. Bodyak;Mao Yin;A Novel Antibody–Drug Conjugate Platform Featuring High Drug Loading and a Controlled Bystander Effect
View the Knowledge base of Antibody-drug Conjugate (ADC):
– What is antibody-drug conjugate (ADC)?
– Antibody-drug conjugate (ADC) in clinical application (Approved/BLA, phaseI/II/III)
– Main elements of antibody-drug conjugate (ADC): Antibodies and their targets
– Main elements of antibody-drug conjugate (ADC):Linker (cleavable/non-cleavable, structure and mechanism)
– Main elements of antibody-drug conjugate (ADC):Toxins/Payloads (Classification and function)
– Toxins/Payloads (Classification and function) of Microtubule destroying drug
– Toxins/Payloads (Classification and function) of DNA damage drugs
– Toxins/Payloads (Classification and function) of Innovative drugs
– Biological coupling technology Chemical based specific in situ antibody modification
– Endogenous coupling of amino acids and Disulfide re bridging strategy
– Glycan coupling
– Site specific biological coupling of engineered antibodies and Enzymatic method
– Biological coupling with engineered unnatural amino acids
– Review for ADC production, quality control and functional assay
– Product data of ADC